Base editing is a groundbreaking genetic technology that allows for precise alterations at the molecular level, offering a promising avenue for correcting genetic disorders. Unlike traditional gene editing techniques that rely on inducing double-strand breaks in DNA, base editing enables direct, irreversible conversion of one DNA base pair into another without breaking the DNA strand. This method significantly reduces the risk of unintended mutations and provides a more controlled approach to gene editing.
To understand how base editing works, it's essential to comprehend the basic building blocks of DNA. DNA is composed of four nucleotides, which are adenine (A), cytosine (C), guanine (G), and thymine (T). These nucleotides pair up in a specific manner: A with T and C with G. Genetic information is encoded in the sequence of these base pairs. However, even a single nucleotide error can lead to genetic disorders, making precision in editing critical.
Base editing typically employs an engineered form of the
CRISPR-Cas9 system. In traditional CRISPR-Cas9 gene editing, an endonuclease enzyme called Cas9 creates double-strand breaks at specific sites in the DNA, guided by a sequence of RNA. The cell's natural repair mechanisms then attempt to fix these breaks, which can be harnessed to introduce desired changes. However, this process is prone to errors, potentially causing unintended mutations.
In contrast, base editing uses a modified form of CRISPR that does not induce double-strand breaks. The base editor consists of a catalytically impaired Cas9 enzyme, meaning it can still target specific DNA sequences but cannot cut the DNA. This modified Cas9 is fused to an enzyme that can chemically modify individual DNA bases. For example, cytosine base editors convert a C-G base pair into a T-A pair by deaminating cytosine to uracil, which is then recognized and replaced by the cell's repair machinery with thymine. Adenine base editors work similarly, converting A-T base pairs to G-C pairs.
This precision is achieved through meticulous design and engineering of the guide RNA and the base editing enzymes to recognize specific target sequences. The process ensures only the intended base pair is altered, minimizing the risk of off-target effects. Additionally, because base editing does not rely on double-strand breaks, it reduces the likelihood of insertions, deletions, or chromosomal rearrangements that can occur with traditional CRISPR techniques.
The implications of base editing are profound. It holds potential for treating a wide range of genetic disorders caused by point mutations, which constitute a significant proportion of known genetic diseases. For example, base editing has shown promise in correcting mutations responsible for conditions such as
sickle cell anemia,
cystic fibrosis, and certain forms of
muscular dystrophy.
Moreover, the technology offers new avenues for developing therapies for complex diseases and advancing synthetic biology. By enabling precise, predictable changes to genetic material, base editing could revolutionize how we approach genetic research and disease treatment.
In conclusion, base editing represents a significant advancement in genetic engineering. Its ability to correct single nucleotide errors without introducing breaks in the DNA makes it a safer and more efficient alternative to traditional gene-editing techniques. As research progresses, base editing could become an integral tool in the fight against genetic diseases, offering hope for effective treatments and potential cures.
For an experience with the large-scale biopharmaceutical model Hiro-LS, please click here for a quick and free trial of its features!
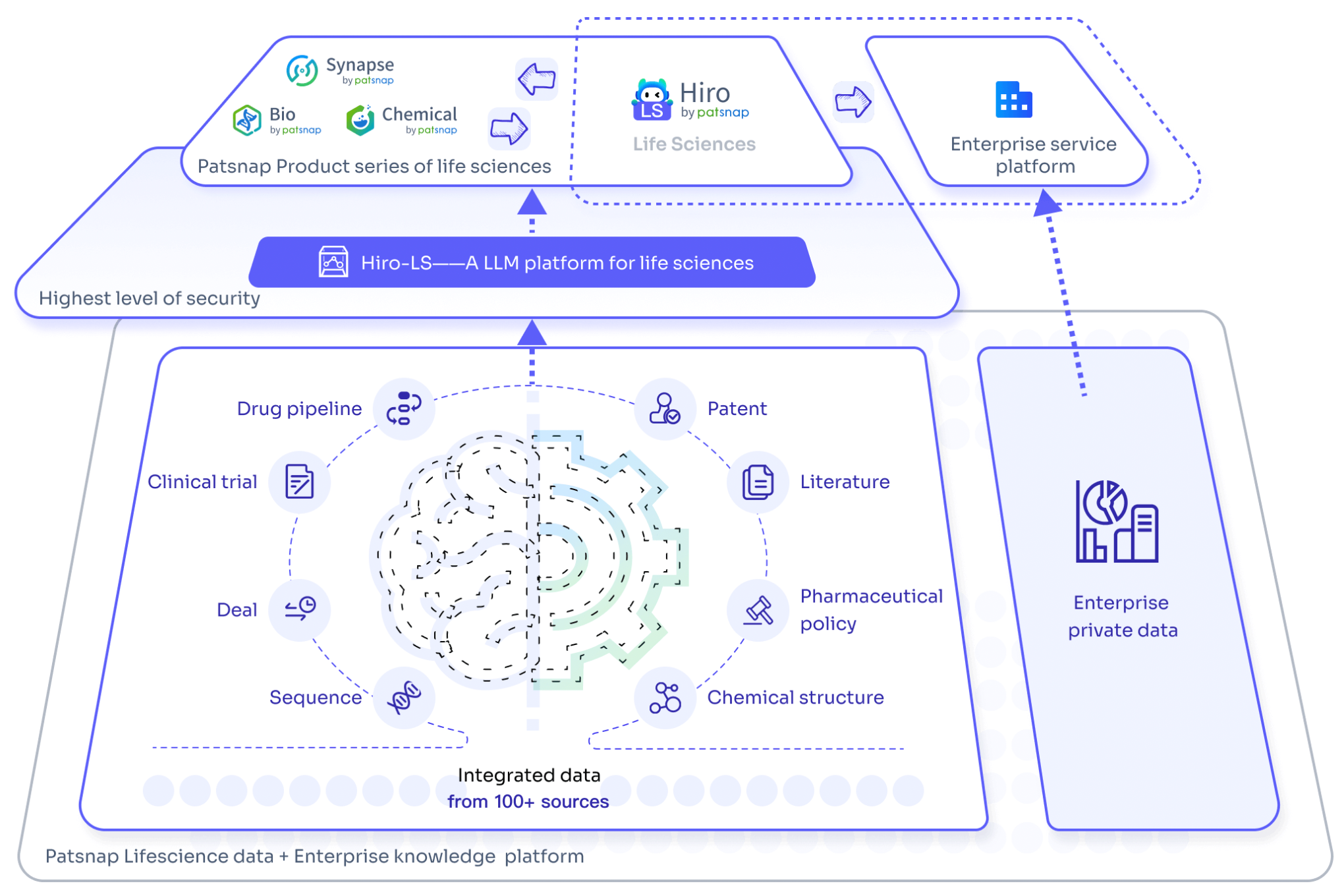