CRISPR, which stands for Clustered Regularly Interspaced Short Palindromic Repeats, is a revolutionary technology that has gained significant attention due to its potential to edit genes with high precision. Originally discovered in the adaptive immune systems of bacteria and archaea, CRISPR plays a crucial role in protecting these organisms from
viral infections. Understanding how CRISPR functions in bacterial systems not only provides insight into bacterial defense mechanisms but also lays the foundation for its application in genetic engineering.
At its core, the CRISPR system is a sophisticated method of storing and utilizing genetic information to recognize and combat viral invaders. When bacteria are attacked by viruses, they have limited options for defense. However, some bacteria have evolved the CRISPR-Cas system, which allows them to acquire snippets of viral DNA and integrate these sequences into their own genome as "spacers" within the CRISPR array. This array acts as a genetic memory bank, documenting past viral threats.
The CRISPR array is composed of repeating sequences, interspersed with these viral-derived spacers. When a bacterium encounters a virus again, it transcribes the CRISPR array into RNA. The RNA is processed into smaller pieces called CRISPR RNAs (crRNAs), each containing a spacer sequence. These crRNAs guide
Cas proteins, the molecular scissors of the system, to any foreign DNA matching the spacer sequence. Once the target DNA is identified, the Cas proteins cleave it, rendering the virus harmless.
A crucial component of this system is the Cas9 protein, which is often highlighted due to its prominent role in gene editing applications. Cas9 is an endonuclease that is guided by crRNA to the target DNA. It then creates a double-strand break, effectively destroying the invader. The precision of this targeting mechanism is what makes
CRISPR-Cas9 such a powerful tool, not only for microbial defense but also for biotechnological applications.
The CRISPR system is highly adaptable. Bacteria can acquire new spacers through a process known as "adaptation," where segments of viral DNA are incorporated into the CRISPR array after an initial
infection. This allows the bacterial immune system to evolve and improve over time, offering protection against a wide variety of viral attacks. The diversity and specificity of spacers across different bacterial species highlight the evolutionary arms race between bacteria and their viral predators.
Interestingly, the utility of CRISPR extends beyond its natural role in bacterial immunity. Researchers have harnessed this system for targeted genetic modifications in a wide range of organisms. By designing synthetic RNA guides that match specific DNA sequences, scientists can direct Cas9 to introduce cuts at desired locations within a genome. This capability has opened new avenues in genetic research, agriculture, and medicine, allowing for precise edits to correct genetic disorders, enhance crop resistance, and study gene function.
In summary, CRISPR in bacterial systems is a sophisticated defense mechanism that enables these microorganisms to fend off viral infections by capturing and utilizing viral genetic information. The system's ability to precisely target and cut DNA has been adapted into a groundbreaking tool for genetic engineering, enabling researchers to explore new frontiers in biology and medicine. Understanding the principles of CRISPR in its natural context not only deepens our knowledge of microbial biology but also enhances our ability to innovate in the field of genetic manipulation.
For an experience with the large-scale biopharmaceutical model Hiro-LS, please click here for a quick and free trial of its features!
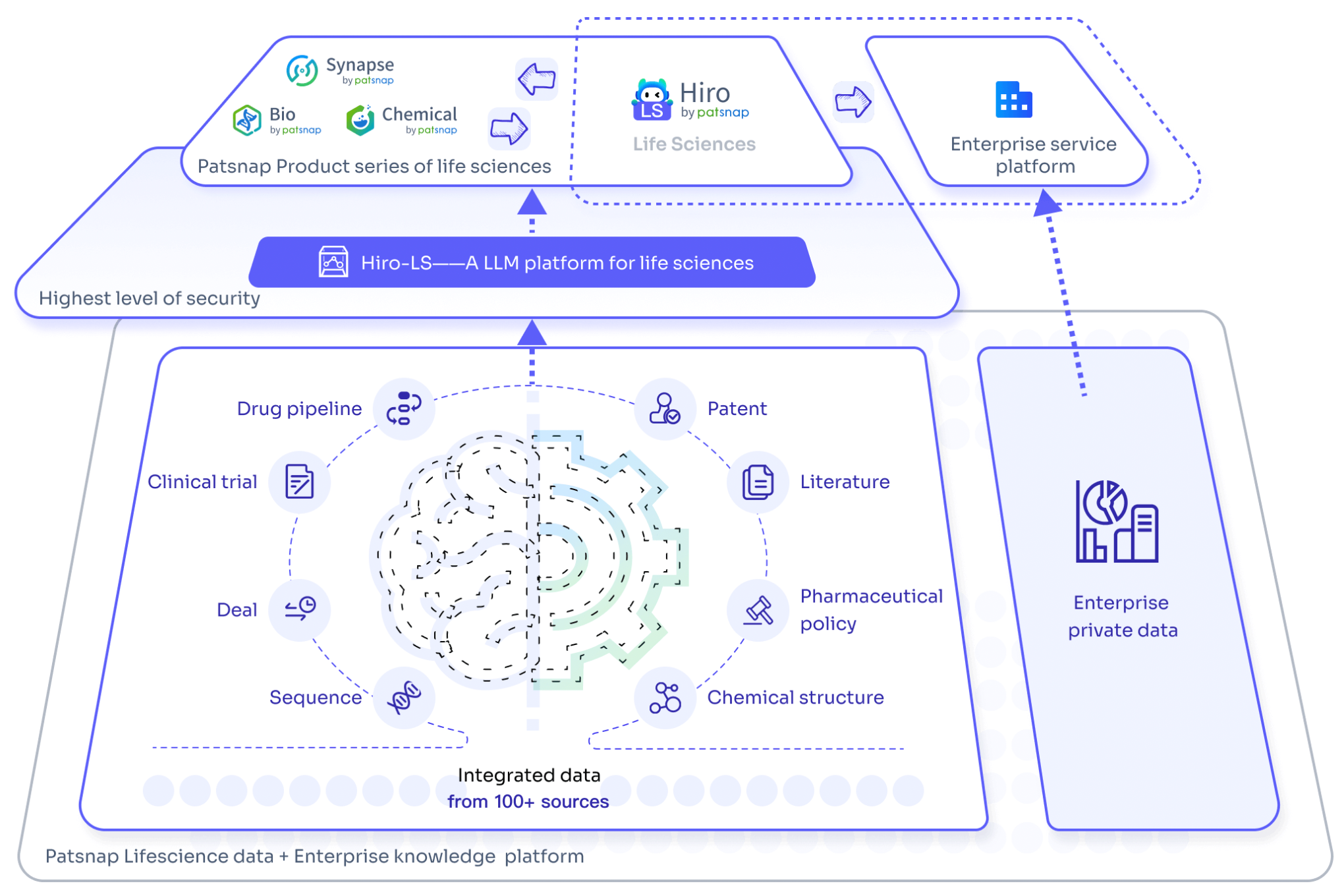