FLAG-tag technology has become a cornerstone in molecular biology and biochemistry for the purification and detection of recombinant proteins. At the heart of its success lies the specific interaction between the FLAG-tag and its corresponding antibody. Understanding the chemistry behind this binding specificity can illuminate why this method is both effective and widely used.
The FLAG-tag itself is a short, hydrophilic peptide sequence composed of eight amino acids: Asp-Tyr-Lys-Asp-Asp-Asp-Asp-Lys (DYKDDDDK). This sequence was designed to be highly antigenic, allowing for strong and specific antibody recognition. The small size of the tag minimizes its impact on the protein to which it is attached, reducing the likelihood of altering the protein's natural function or structure.
The binding specificity between the FLAG-tag and the FLAG antibody, often an anti-FLAG M2 monoclonal antibody, is primarily driven by the precise interaction between the amino acid residues of the FLAG sequence and the complementary binding sites on the antibody. Antibodies recognize specific epitopes, and in this case, the DYKDDDDK sequence acts as a distinct epitope. The negatively charged aspartic acid residues (Asp) play a crucial role in forming electrostatic interactions with positively charged residues located within the antibody’s paratope, the part of the antibody that binds to the antigen.
Furthermore, the tyrosine (Tyr) and lysine (Lys) residues contribute to binding through additional non-covalent interactions such as hydrogen bonding and van der Waals forces. These interactions are essential for the stability and specificity of the antigen-antibody complex. The lysine residue, in particular, helps to stabilize the interaction through hydrogen bonds with amino acid residues on the antibody that can act as hydrogen bonding partners.
The design of the FLAG-tag also takes advantage of steric factors. The spatial arrangement of the amino acids in the FLAG sequence ensures that the epitope is accessible to the antibody, minimizing steric hindrance that could otherwise impede binding. This accessibility is crucial for both the binding strength and the ease with which the antibody can recognize the FLAG-tag in various experimental conditions, including those involving complex protein mixtures.
Additionally, the FLAG-tag’s hydrophilic nature ensures that it remains exposed on the surface of proteins in aqueous environments, which is a common condition in biological assays. This exposure further aids in the efficient binding of the anti-FLAG antibody, as it can readily access and bind to the epitope without significant interference from the protein structure.
In practical applications, the high specificity and affinity of the FLAG-tag/antibody interaction facilitate diverse experimental techniques, including western blotting, immunoprecipitation, and affinity chromatography. These methods benefit from the tag’s ability to provide a consistent and reliable means of detecting and purifying tagged proteins, critical for experimental reproducibility and accuracy.
In summary, the chemistry underlying the binding specificity of FLAG-tag antibodies is a finely tuned interplay of electrostatic interactions, hydrogen bonding, van der Waals forces, and steric accessibility. This sophisticated balance ensures that the FLAG-tag technology remains a powerful tool in the study and manipulation of proteins, helping researchers to unlock the mysteries of cellular biology. The ingenuity of its design highlights the intricate connection between molecular structure and function, showcasing the elegance of biochemical engineering.
For an experience with the large-scale biopharmaceutical model Hiro-LS, please click here for a quick and free trial of its features!
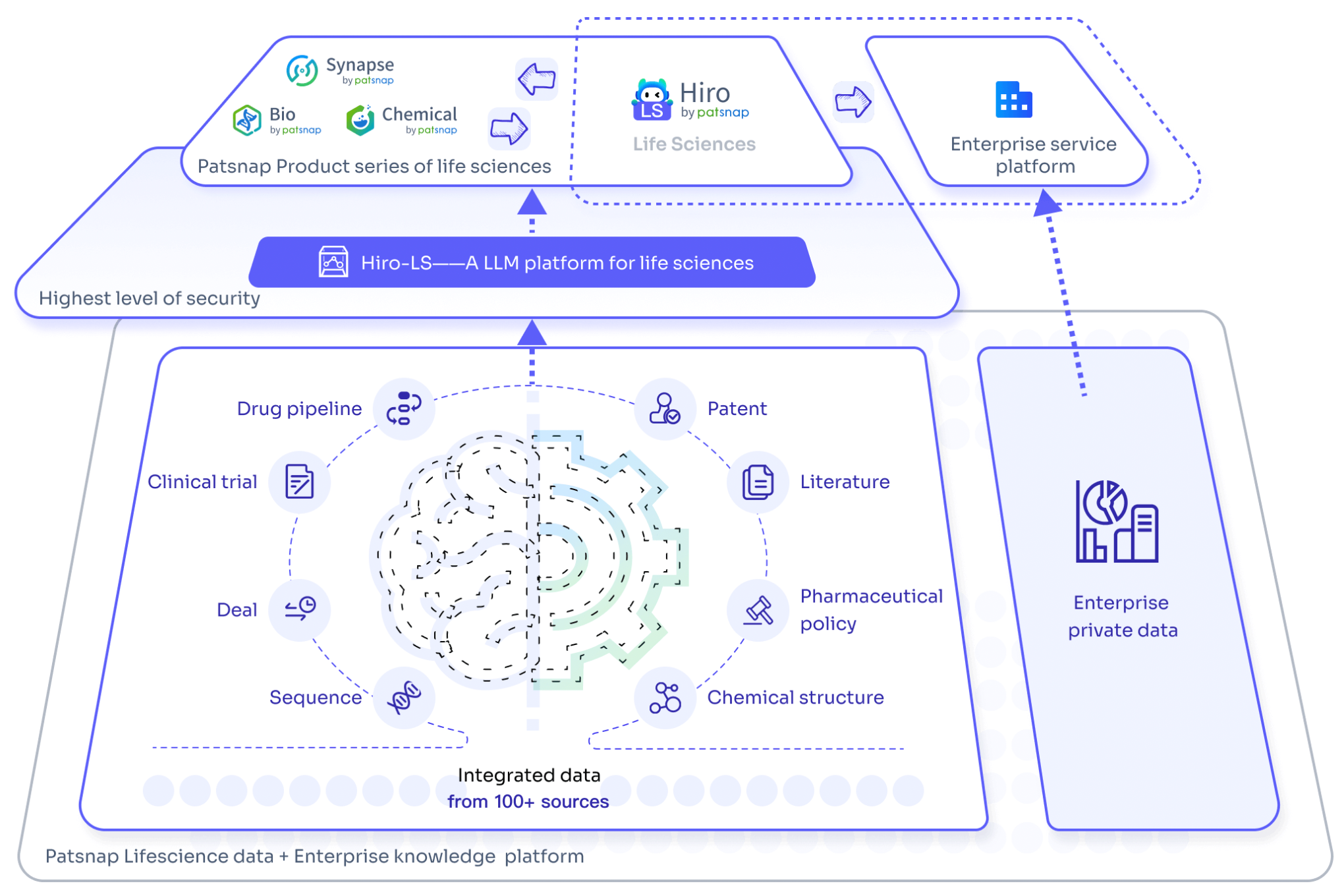