Reverse Transcription quantitative Polymerase Chain Reaction (RT-qPCR) is a powerful and sensitive technique used to quantify RNA levels in a sample. This method combines reverse transcription of RNA into complementary DNA (cDNA) with quantitative PCR, allowing researchers to measure gene expression with accuracy and precision. Understanding the key steps in the RT-qPCR workflow is crucial for obtaining reliable and reproducible results. Below, we delve into the essential stages involved in this process.
The first step in the RT-qPCR workflow is RNA extraction. This step is critical because the quality and purity of the RNA can significantly affect the reliability of the results. High-quality RNA should be free from contaminants such as proteins, DNA, and enzymatic inhibitors. Several commercial kits and methods are available for isolating RNA, and the choice often depends on the type of sample and the downstream applications. It is imperative to work quickly and efficiently during RNA extraction to prevent degradation of RNA by ribonucleases (RNases). Verifying RNA integrity through techniques like agarose gel electrophoresis or using a spectrophotometer to check purity ratios can help ensure the success of subsequent steps.
Once high-quality RNA is obtained, the next step is reverse transcription. In this step, RNA is reverse-transcribed into cDNA using reverse transcriptase enzymes. Selecting the appropriate reverse transcription kit or enzyme is essential, as this influences the efficiency and yield of cDNA synthesis. Researchers often use random hexamers, oligo(dT) primers, or gene-specific primers to initiate the reverse transcription process, and each choice has its own advantages depending on the experimental goals.
After generating cDNA, the next phase is the quantitative PCR (qPCR) itself. This involves amplifying the cDNA and measuring the amount of PCR product in real-time using fluorescent dyes or probes. The PCR mix typically contains DNA polymerase, deoxynucleotide triphosphates (dNTPs), primers, and a fluorescent dye such as SYBR Green or TaqMan probes. The choice between SYBR Green and probe-based chemistry depends on factors such as specificity and budget. Precise primer design is crucial to ensure specificity and efficiency during amplification, preventing nonspecific amplification and primer-dimer formations, which can skew results.
The qPCR run is conducted in a thermocycler equipped with a fluorescence detection system. The process involves denaturation, annealing, and extension steps, which are repeated for 30-40 cycles. During each cycle, the increase in fluorescence signal is proportional to the amount of amplified product, allowing quantification of initial RNA levels. It’s essential to include appropriate controls, such as no-template controls and no-reverse-transcription controls, to identify any potential contamination or amplification of genomic DNA.
Data analysis is the final critical step in the RT-qPCR workflow. The threshold cycle (Ct) value, which represents the cycle number at which the fluorescence exceeds a set threshold, is used to quantify the initial amount of target RNA. Relative quantification often involves comparing Ct values between samples and a reference gene to normalize for sample variation. Absolute quantification requires generating a standard curve with known concentrations of the target sequence. Careful analysis and interpretation of the data ensure that the results are meaningful and reliable.
In conclusion, the RT-qPCR workflow is a meticulous process that requires attention to detail at every stage, from RNA extraction to data analysis. By understanding and carefully executing each step, researchers can achieve accurate quantification of gene expression, contributing valuable insights into biological processes and disease mechanisms.
For an experience with the large-scale biopharmaceutical model Hiro-LS, please click here for a quick and free trial of its features!
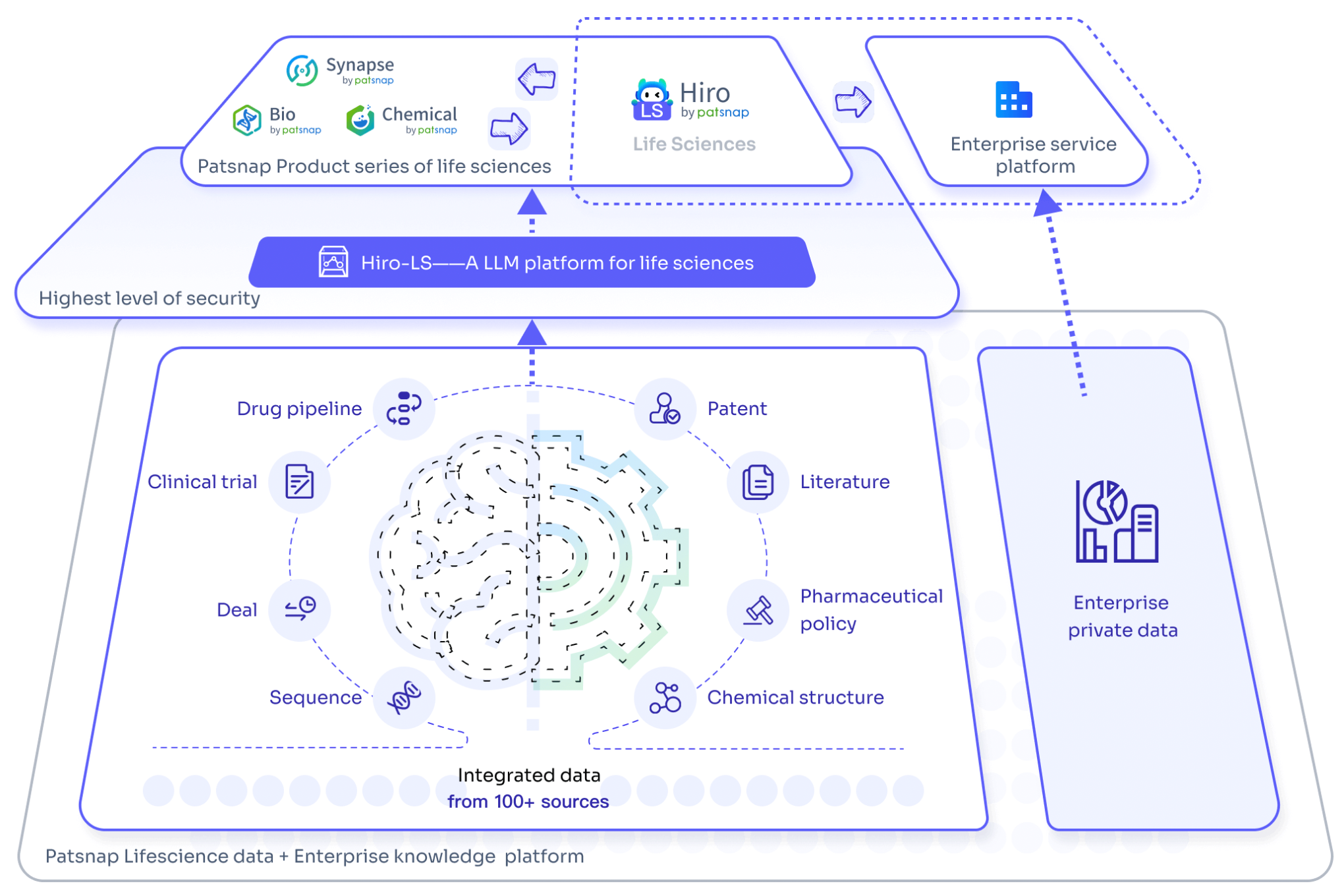