Metabolic engineering is a fascinating field at the intersection of biology, chemistry, and engineering. It involves the purposeful modification of metabolic pathways within an organism to increase the production of specific substances or to endow the organism with new capabilities. By manipulating the metabolic processes of microorganisms, plants, or even animals, scientists and engineers can enhance the synthesis of valuable compounds, enable the degradation of unwanted materials, or create entirely new functionalities.
At its core, metabolic engineering relies on a deep understanding of cellular metabolism. Metabolic pathways are a series of chemical reactions occurring within a cell that lead to the formation or transformation of a compound. These pathways are highly regulated and complex, involving numerous enzymes and regulatory proteins. By employing tools like genetic engineering, genome editing, and synthetic biology, scientists can alter these pathways to achieve desired outcomes.
In industry, metabolic engineering has found numerous applications, playing a pivotal role in sectors ranging from pharmaceuticals to agriculture, and even energy. One of its most significant contributions is in the production of biofuels. As the world seeks sustainable alternatives to fossil fuels, biofuels derived from renewable biological resources are gaining traction. Metabolic engineering is employed to optimize microorganisms such as yeast or bacteria to efficiently convert biomass into biofuels like ethanol or biodiesel. This not only enhances yield but also reduces production costs, making biofuels a more viable alternative.
The pharmaceutical industry also benefits immensely from metabolic engineering. Traditionally, many drugs are derived from natural products produced by plants, fungi, or bacteria. Metabolic engineering enables the large-scale production of these bioactive compounds by transferring and optimizing the relevant metabolic pathways in host organisms. For example, the production of the antimalarial drug artemisinin was revolutionized by engineering yeast to produce its precursor, significantly lowering production costs and increasing accessibility.
Another exciting application is in the field of agriculture, where metabolic engineering is used to develop crop varieties with improved traits. By tweaking the metabolic pathways of plants, scientists can enhance nutritional content, increase resistance to pests and diseases, or improve stress tolerance. This biotechnological approach not only supports food security but also promotes sustainable agricultural practices.
In addition to these, metabolic engineering is increasingly being applied to the production of specialty chemicals, flavors, fragrances, and even biodegradable plastics. By engineering microorganisms to produce these compounds, industries can move away from petrochemical processes, reducing environmental impact and reliance on non-renewable resources.
Despite its potential, metabolic engineering does face challenges. The complexity of metabolic networks makes it difficult to predict the outcomes of genetic modifications. Additionally, unintended effects can arise, impacting the growth and viability of the engineered organism. However, advances in computational biology, systems biology, and machine learning are providing new tools and models to better understand and navigate these complexities.
In conclusion, metabolic engineering stands as a cornerstone of modern biotechnology, offering innovative solutions to some of the world's most pressing challenges. Its ability to harness and modify the intricate networks of life not only showcases the marvels of modern science but also paves the way for a more sustainable and prosperous future. As the field continues to evolve, its applications will undoubtedly expand, unlocking new possibilities across various industries.
For an experience with the large-scale biopharmaceutical model Hiro-LS, please click here for a quick and free trial of its features!
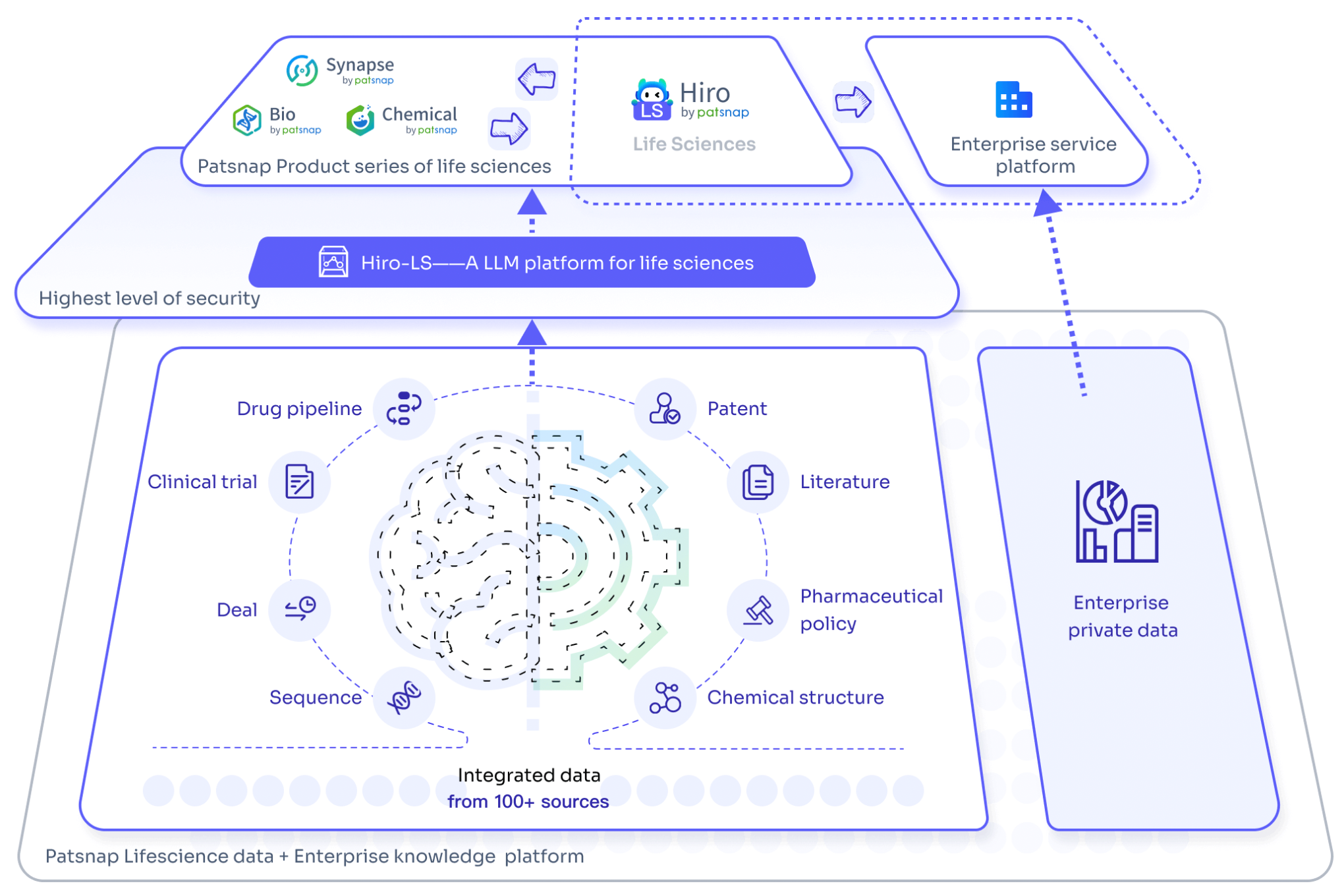