Electron transport complex III inhibitors are a fascinating class of compounds that play a significant role in cellular bioenergetics. To appreciate the importance of these inhibitors, it's essential first to understand the function of Complex III within the electron transport chain (ETC). The ETC is a series of protein complexes located in the inner mitochondrial membrane, crucial for the production of adenosine triphosphate (ATP) through oxidative phosphorylation. Complex III, also known as cytochrome bc1 complex, is the third complex in this chain and is pivotal in maintaining the flow of electrons from ubiquinol to cytochrome c. The inhibition of this complex disrupts the electron transport process, leading to various biochemical and physiological consequences.
Electron transport complex III inhibitors function by binding to specific sites within the cytochrome bc1 complex, thereby interrupting the normal passage of electrons. This blockade prevents the reduction of
cytochrome c and halts the transfer of electrons to Complex IV. As a result, the proton gradient across the inner mitochondrial membrane diminishes, impairing the mitochondrion's ability to produce ATP. Additionally, this disruption can lead to the leakage of electrons and the formation of reactive oxygen species (ROS), which can cause oxidative damage to cellular components.
There are several types of Complex III inhibitors, each targeting different binding sites within the cytochrome bc1 complex. The most well-known inhibitor is antimycin A, a natural product that binds to the Qi site, inhibiting the oxidation of ubiquinol. Stigmatellin and myxothiazol are other examples that bind to the Qo site, preventing the reduction of
cytochrome c1. By targeting distinct sites, these inhibitors provide valuable tools for studying the mechanistic aspects of electron transport and the structural biology of Complex III.
The clinical and research applications of Electron transport complex III inhibitors are diverse. In research settings, these inhibitors are instrumental in elucidating the mechanisms of the electron transport chain and mitochondrial bioenergetics. By selectively blocking Complex III, researchers can study the resulting changes in cellular metabolism, ROS production, and ATP synthesis. This knowledge is crucial for understanding the pathophysiology of
mitochondrial diseases and for developing potential therapeutic strategies.
In a medical context, Complex III inhibitors have shown promise in the treatment of
parasitic diseases. For instance,
atovaquone, a well-known Complex III inhibitor, is used as an antimalarial agent. It targets the cytochrome bc1 complex in the mitochondria of Plasmodium falciparum, the parasite responsible for
malaria, thereby disrupting its energy metabolism and leading to parasite death. Similarly, atovaquone has been employed to treat
Pneumocystis pneumonia, a
severe infection in immunocompromised patients, by inhibiting the energy production in Pneumocystis jirovecii.
Moreover, Complex III inhibitors have potential applications in
cancer therapy. Cancer cells often exhibit altered metabolic pathways and a reliance on mitochondrial function for survival and proliferation. By inhibiting Complex III, it is possible to exploit the metabolic vulnerabilities of cancer cells, leading to cell death. This approach is being actively explored in preclinical studies, aiming to develop novel anticancer therapeutics.
Despite their potential, the use of Electron transport complex III inhibitors in clinical settings is not without challenges. The inhibition of mitochondrial function can have profound effects on normal cells, leading to toxicity and adverse effects. Therefore, it is crucial to develop inhibitors that selectively target diseased cells while sparing healthy tissues. Advances in drug delivery systems and targeted therapies hold promise in addressing these challenges, paving the way for safer and more effective use of Complex III inhibitors.
In conclusion, Electron transport complex III inhibitors are powerful tools with significant implications for research and medicine. By disrupting the electron transport chain, these inhibitors provide insights into mitochondrial function and offer potential therapeutic avenues for diseases such as malaria, Pneumocystis pneumonia, and cancer. However, the effective and safe application of these inhibitors requires a deep understanding of their mechanisms and careful consideration of their potential impacts on cellular physiology. As research progresses, the future holds great promise for the development of sophisticated strategies to harness the power of Complex III inhibitors in improving human health.
How to obtain the latest development progress of all targets?
In the Synapse database, you can stay updated on the latest research and development advances of all targets. This service is accessible anytime and anywhere, with updates available daily or weekly. Use the "Set Alert" function to stay informed. Click on the image below to embark on a brand new journey of drug discovery!
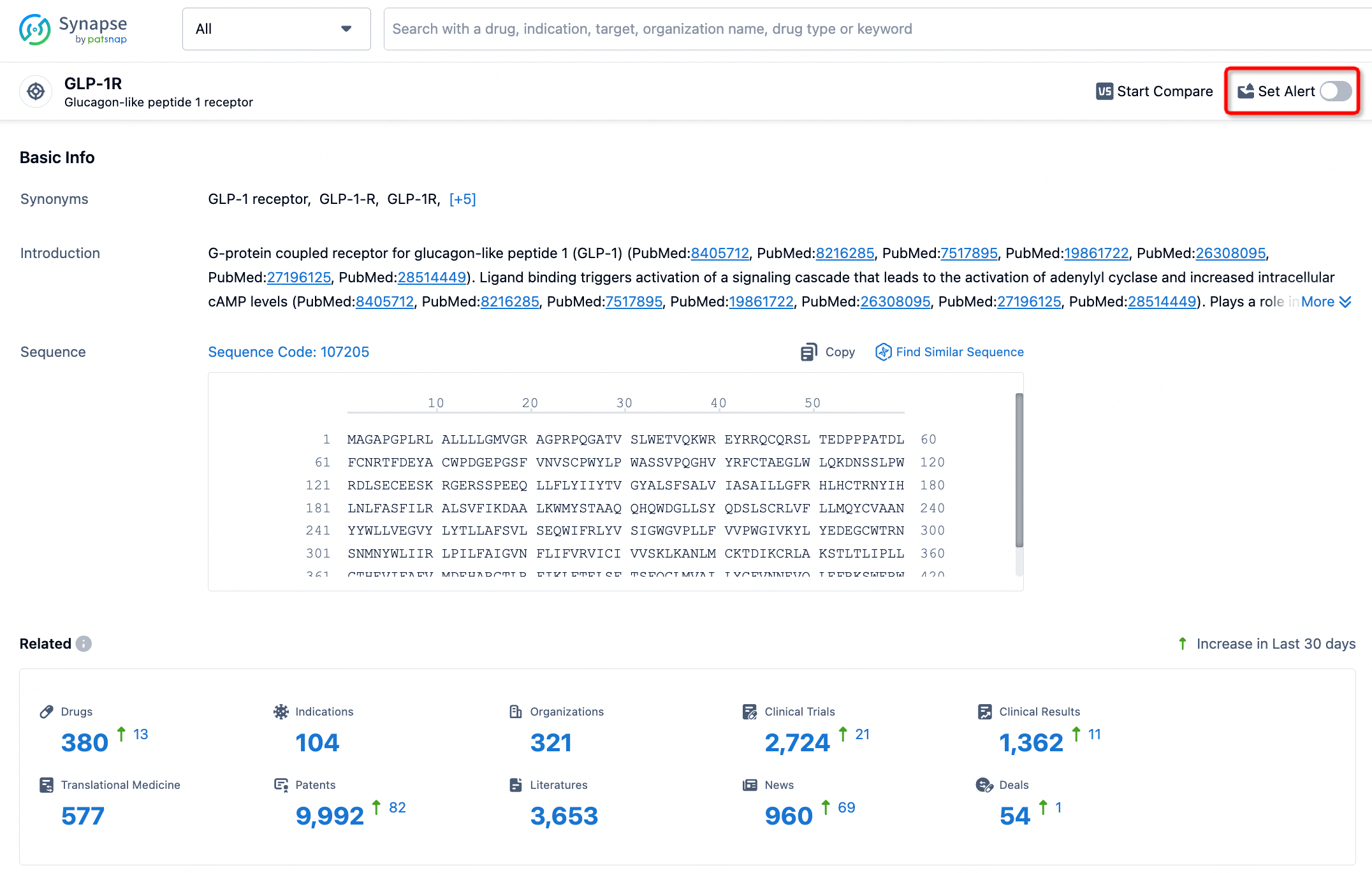
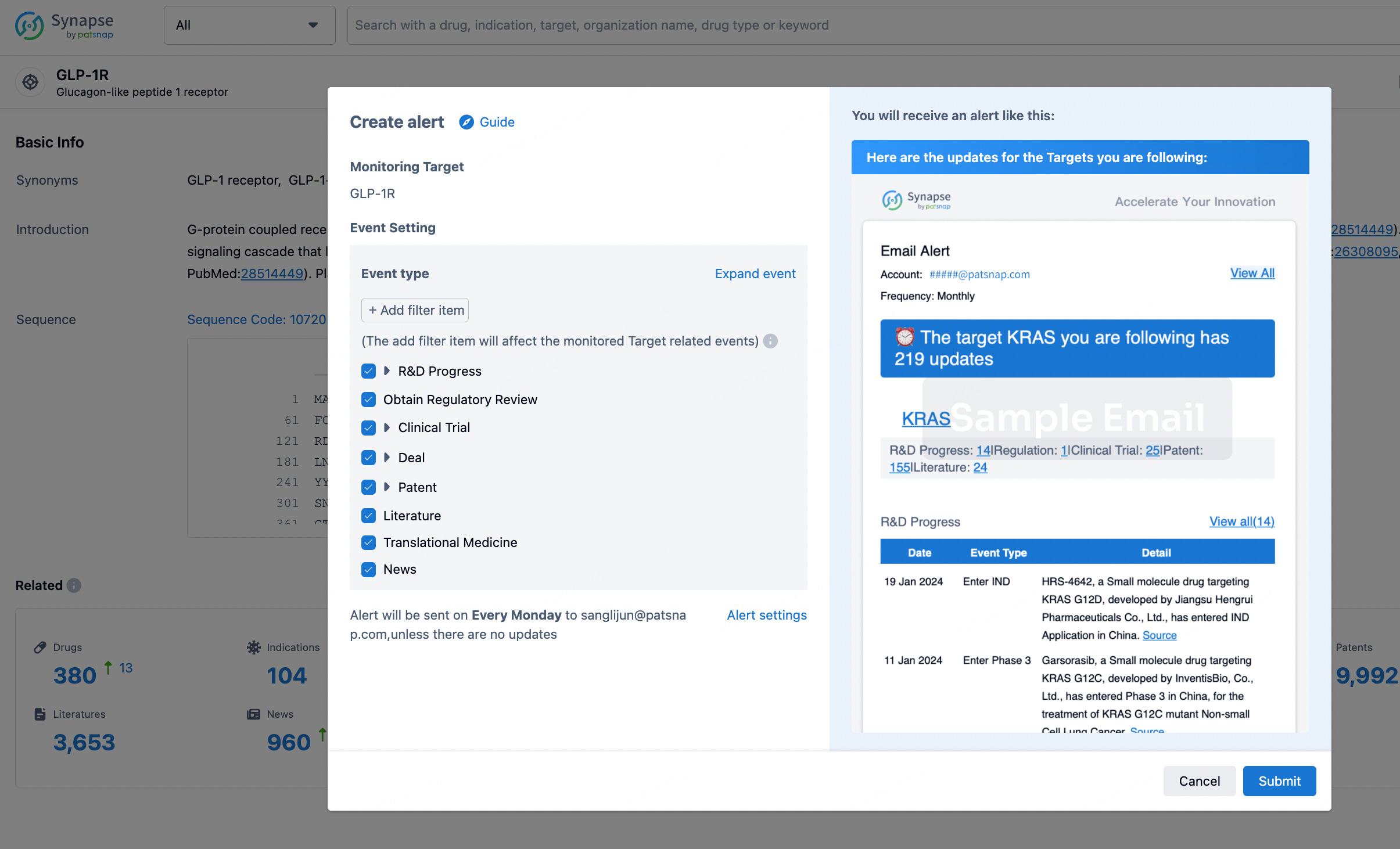
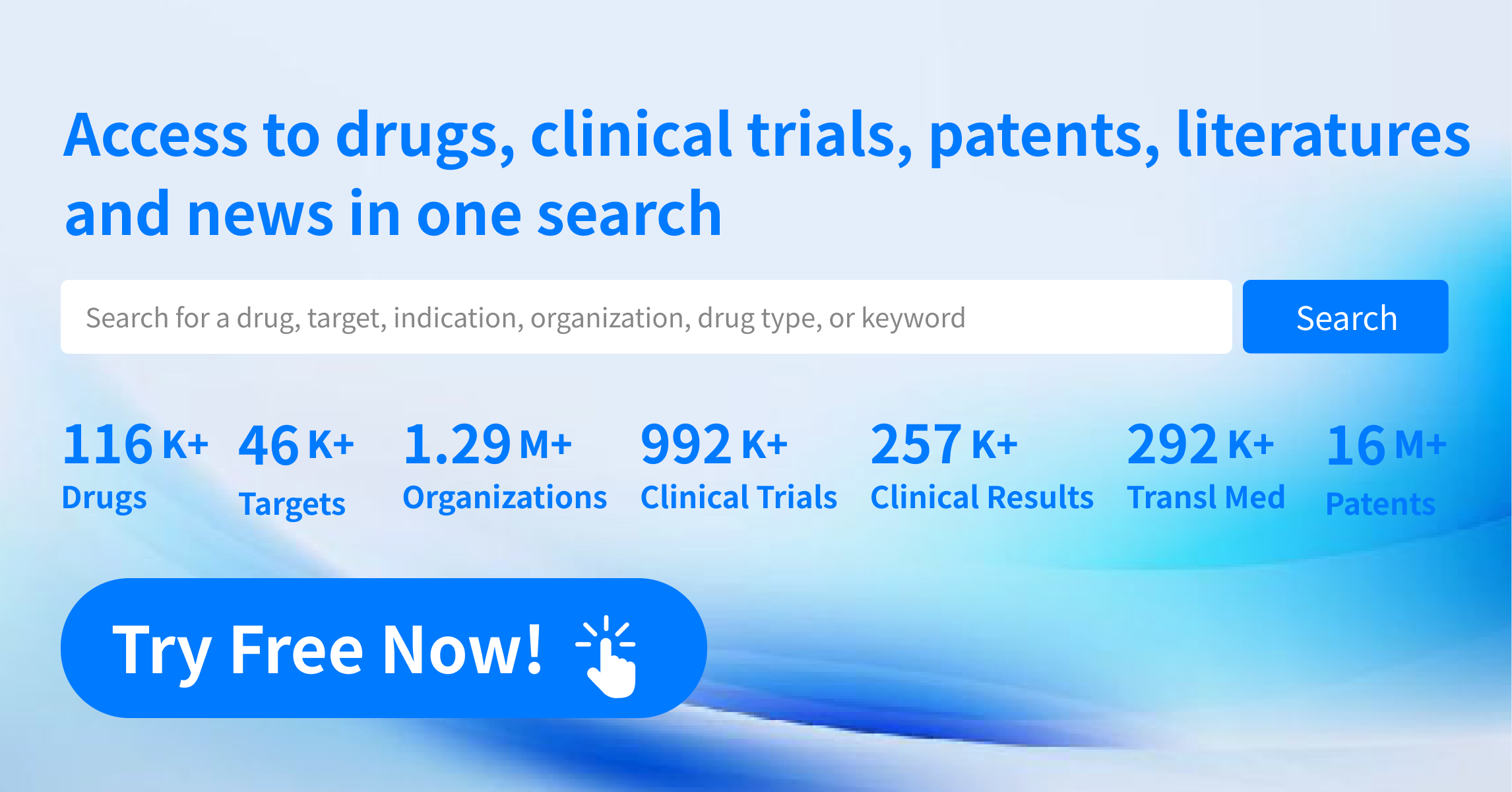