Mitochondrial complex I, also known as NADH dehydrogenase, plays a crucial role in cellular respiration, which is the process by which cells generate energy in the form of adenosine triphosphate (ATP). Located in the inner mitochondrial membrane, complex I is the first enzyme of the mitochondrial electron transport chain (ETC). It catalyzes the transfer of electrons from NADH to ubiquinone, coupled with the translocation of protons across the mitochondrial membrane. This process is fundamental for the establishment of the proton gradient that drives ATP synthesis.
Given the pivotal role of mitochondrial complex I in cellular bioenergetics, inhibitors of this enzyme can have profound effects on the physiology of cells. Mitochondrial complex I inhibitors are a diverse group of substances that obstruct the normal function of the enzyme, leading to a cascade of cellular events. These inhibitors can be either naturally occurring or synthetic and can have therapeutic as well as toxicological significance.
Mitochondrial complex I inhibitors work by binding to specific sites on the NADH dehydrogenase enzyme, thereby impeding the transfer of electrons from NADH to ubiquinone. This interruption halts the entire electron transport chain, preventing the establishment of the proton gradient essential for ATP synthesis. Consequently, the cell's energy production is severely compromised. Depending on the inhibitor and its binding site, the inhibition can be either reversible or irreversible.
The mechanism of inhibition can vary depending on the specific inhibitor. For example,
rotenone, a well-known plant-derived inhibitor, binds to the ubiquinone binding site, blocking electron transfer. Another inhibitor, piericidin A, mimics the structure of
ubiquinone and competes for its binding site. These inhibitors essentially "clog" the electron transport system, leading to the accumulation of NADH and a decrease in ATP production. Consequently, cells may resort to anaerobic metabolism, leading to the production of lactate and subsequent
cellular acidosis.
In addition to direct inhibition, some complex I inhibitors can also induce reactive oxygen species (ROS) production by increasing electron leakage from the electron transport chain. This oxidative stress can lead to cellular damage and has been implicated in the pathogenesis of various diseases, including
neurodegenerative disorders and
ischemia-reperfusion injury.
Mitochondrial complex I inhibitors have a range of applications, from research tools to potential therapeutic agents. In the laboratory, these inhibitors are extensively used to study mitochondrial function and the bioenergetics of cells. By selectively inhibiting complex I, researchers can investigate the role of
mitochondrial dysfunction in various diseases and identify potential therapeutic targets.
One of the most promising therapeutic applications of complex I inhibitors is in
cancer treatment. Cancer cells often exhibit altered metabolism, known as the Warburg effect, relying heavily on glycolysis for energy production even in the presence of oxygen. By inhibiting complex I, it is possible to selectively target the metabolic flexibility of cancer cells, thereby sensitizing them to apoptosis. Several complex I inhibitors are currently under investigation for their potential use as anti-cancer agents.
In addition to cancer, complex I inhibitors have been explored in the context of neurodegenerative diseases such as
Parkinson's disease. Rotenone, despite its toxicity, has been used to create animal models of Parkinson's disease, providing insights into the role of mitochondrial dysfunction in its pathogenesis. Understanding how complex I inhibitors induce neurodegeneration can help in identifying potential therapeutic strategies to counteract these effects.
Lastly, some mitochondrial complex I inhibitors have practical applications as insecticides and pesticides. Rotenone, for instance, has been used in agriculture to control insect populations. However, due to its toxicity to humans and other non-target species, its use has become increasingly restricted.
In conclusion, mitochondrial complex I inhibitors are a powerful tool in both research and therapeutic contexts. By disrupting the normal function of NADH dehydrogenase, these inhibitors provide insights into mitochondrial biology and offer potential avenues for the treatment of various diseases. However, their use must be carefully managed due to the potential for significant cellular and physiological disruption.
How to obtain the latest development progress of all targets?
In the Synapse database, you can stay updated on the latest research and development advances of all targets. This service is accessible anytime and anywhere, with updates available daily or weekly. Use the "Set Alert" function to stay informed. Click on the image below to embark on a brand new journey of drug discovery!
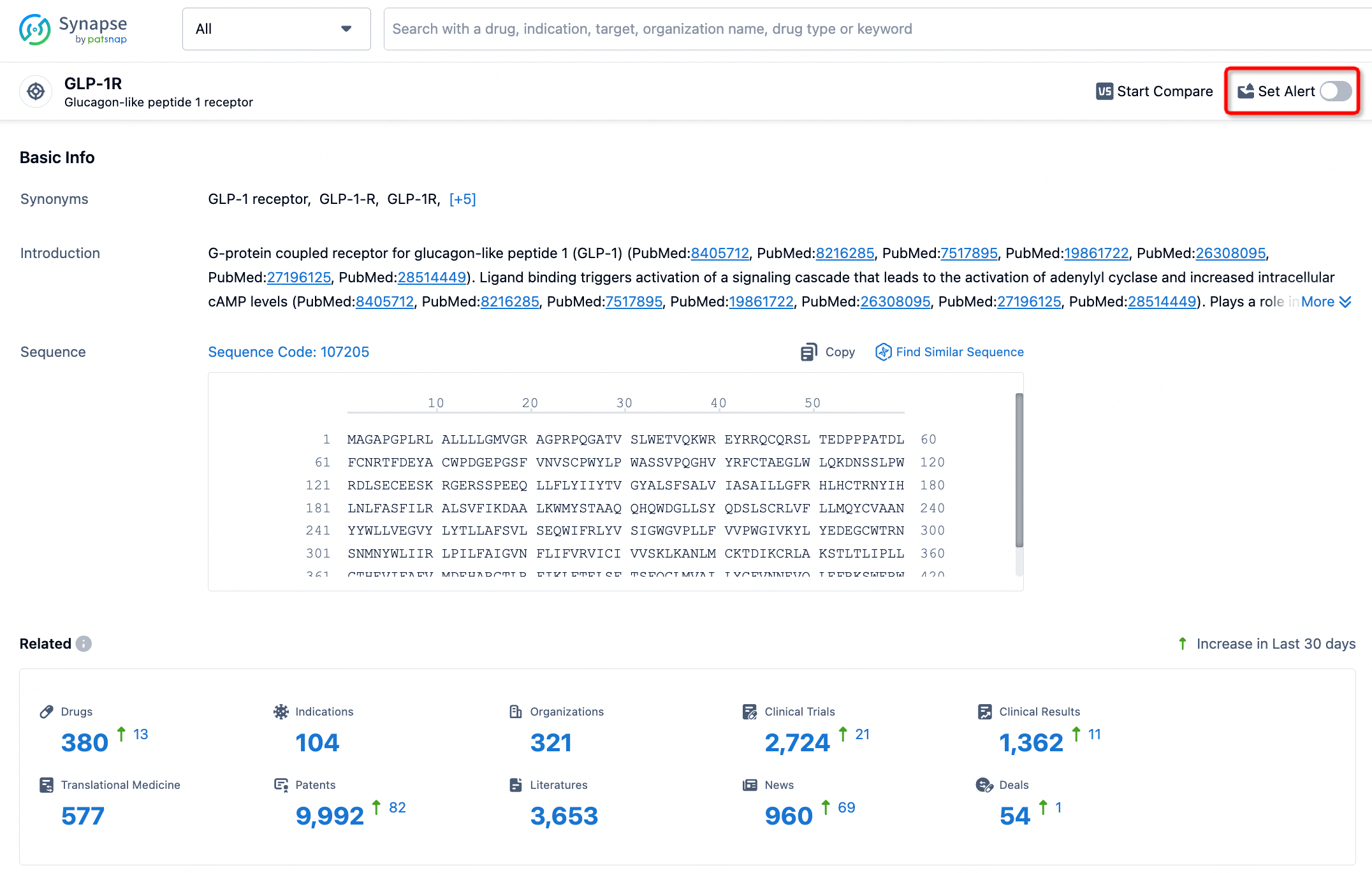
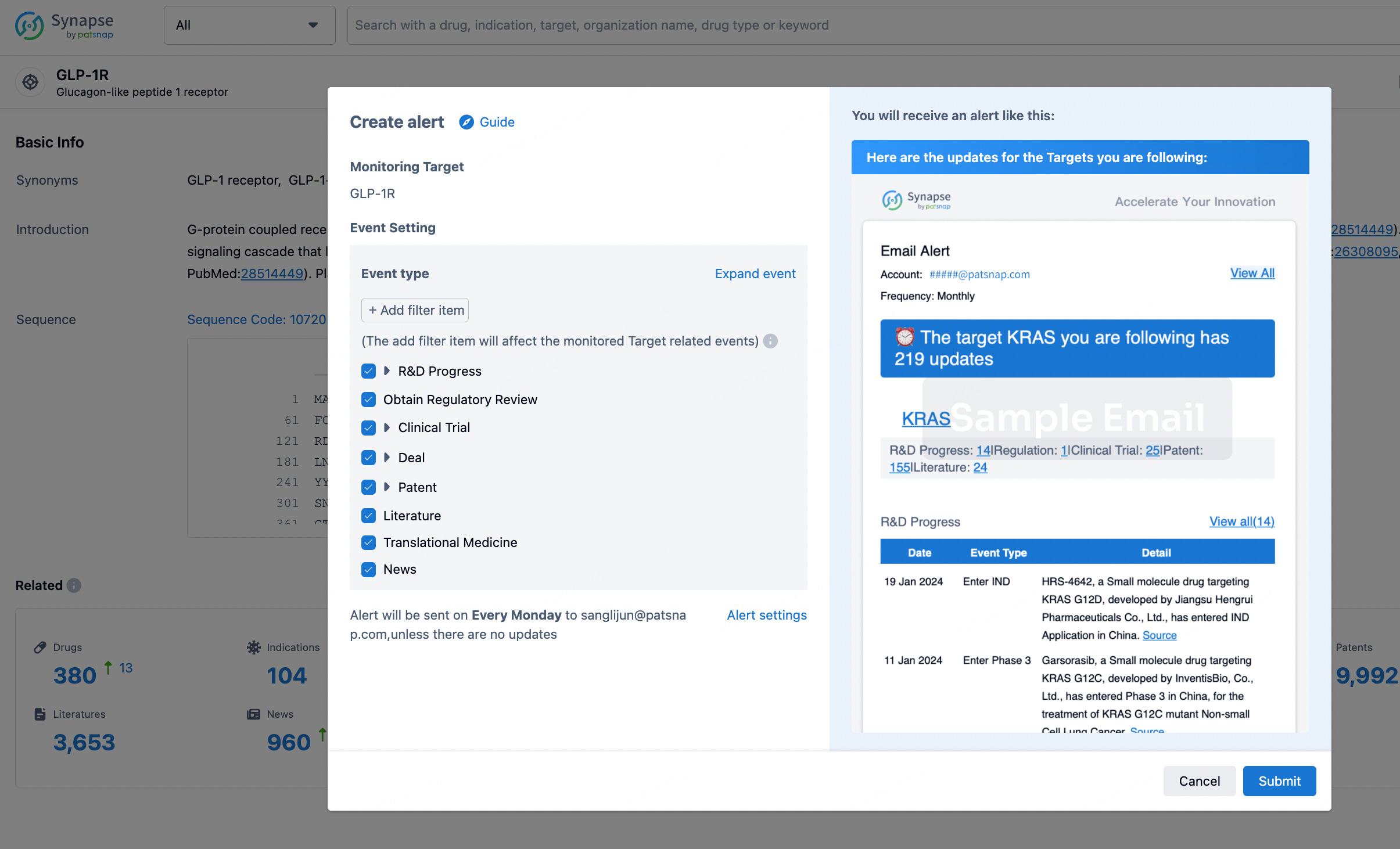
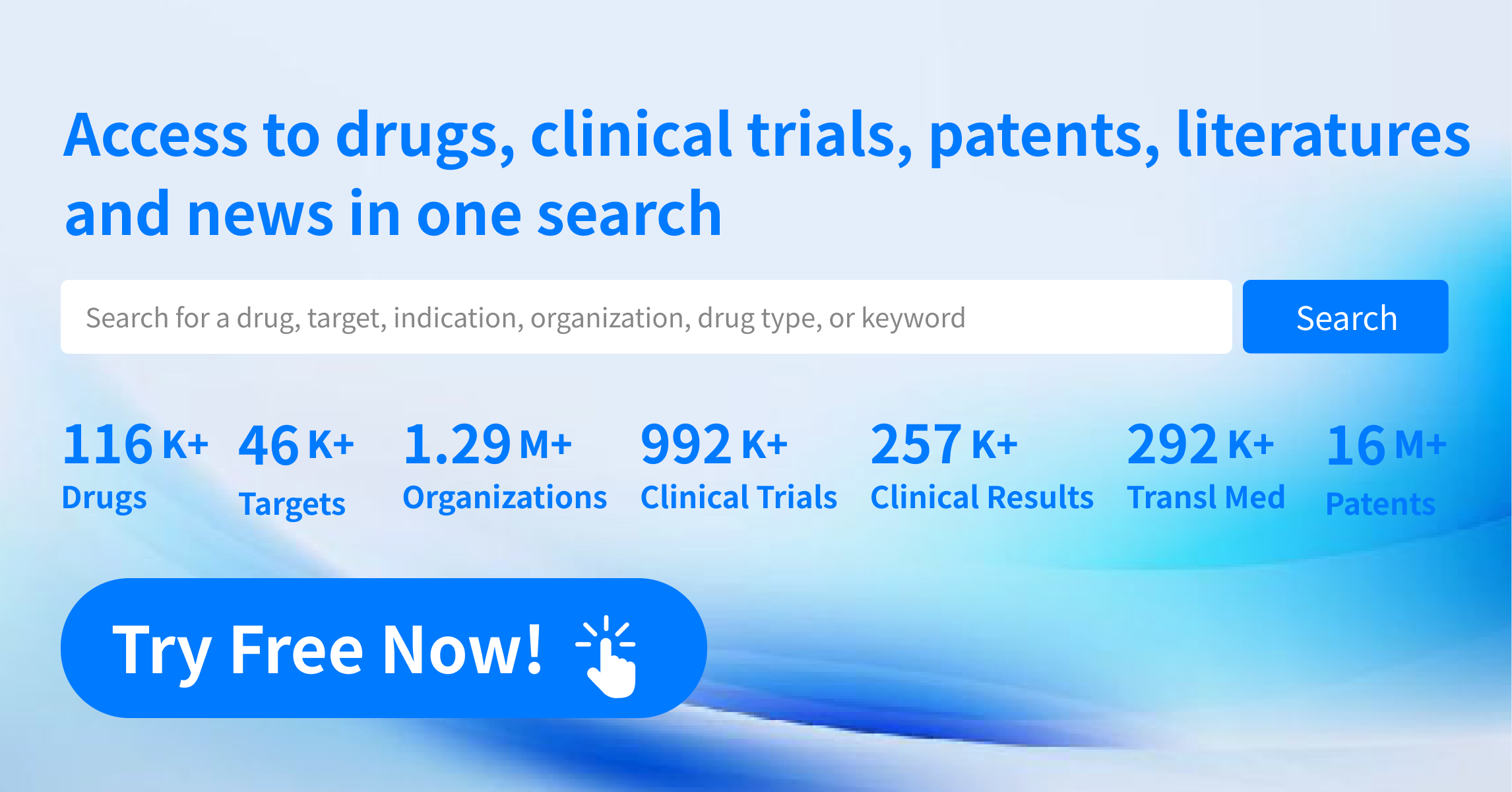