Short hairpin RNA (shRNA) is a powerful tool in the field of genetic research, particularly for its ability to provide long-term gene silencing. This process is crucial for studying gene function, understanding diseases, and developing novel therapeutic approaches. Understanding shRNA and its mechanism provides insight into how scientists can manipulate gene expression in a controlled and sustained manner.
At its core, shRNA is a sequence of RNA that, once introduced into a cell, forms a tight hairpin turn. Typically, shRNA is encoded within a DNA vector and is transcribed inside the target cell. The structure of the shRNA includes a stem-loop motif, which is crucial for its function. The stem consists of two complementary RNA sequences that hybridize to form a double-stranded region, while the loop connects the stem's two ends.
Upon transcription, shRNA is processed by the cellular machinery. The enzyme
Dicer, a ribonuclease in the RNA interference (RNAi) pathway, recognizes the hairpin structure and cleaves it into smaller double-stranded RNA fragments. These fragments are then incorporated into the RNA-induced silencing complex (RISC). Within RISC, one strand of the RNA duplex, known as the guide strand, is retained while the other strand is degraded. The guide strand directs
RISC to the complementary messenger RNA (mRNA) target.
Once bound to the target mRNA, RISC facilitates its degradation or represses its translation, effectively silencing the gene. This post-transcriptional silencing mechanism makes shRNA a valuable tool for researchers aiming to knockdown specific genes. Unlike transient methods of gene silencing, such as small interfering RNA (siRNA), shRNA offers the advantage of potentially long-term gene suppression. This is largely due to its stable expression from the integrated DNA vector, which allows for continuous production of shRNA molecules within the cell.
The ability to achieve long-term gene silencing with shRNA has significant implications. In research, it enables scientists to study the effects of sustained gene knockdown on cellular processes and organismal development. It is particularly useful in the study of diseases where chronic gene suppression can mimic
pathological conditions, providing insights into disease mechanisms and potential treatment strategies.
Moreover, shRNA has promising therapeutic applications. By targeting genes involved in disease pathways, shRNA-based therapies have the potential to provide lasting treatment effects. For instance, in
cancer research, shRNA can be used to target oncogenes, genes that, when mutated or overexpressed, drive the progression of cancer. Silencing these genes can inhibit tumor growth and proliferation. Additionally, shRNA can be engineered to target viral genes, offering a strategy for combating
viral infections.
Despite its potential, the application of shRNA technology also faces challenges. Delivering shRNA vectors effectively and safely to target cells in vivo remains a significant hurdle. Researchers are exploring various delivery methods, such as viral vectors and nanoparticles, to overcome these obstacles. Moreover, ensuring specificity and minimizing off-target effects are critical considerations to prevent unintended gene silencing and adverse effects.
In conclusion, shRNA is a versatile tool that offers the ability to achieve long-term gene silencing. Its role in research and therapeutic development continues to expand as scientists refine delivery methods and enhance specificity. By harnessing the power of shRNA, researchers are uncovering new insights into gene function and paving the way for innovative treatments for a host of genetic and infectious diseases.
For an experience with the large-scale biopharmaceutical model Hiro-LS, please click here for a quick and free trial of its features!
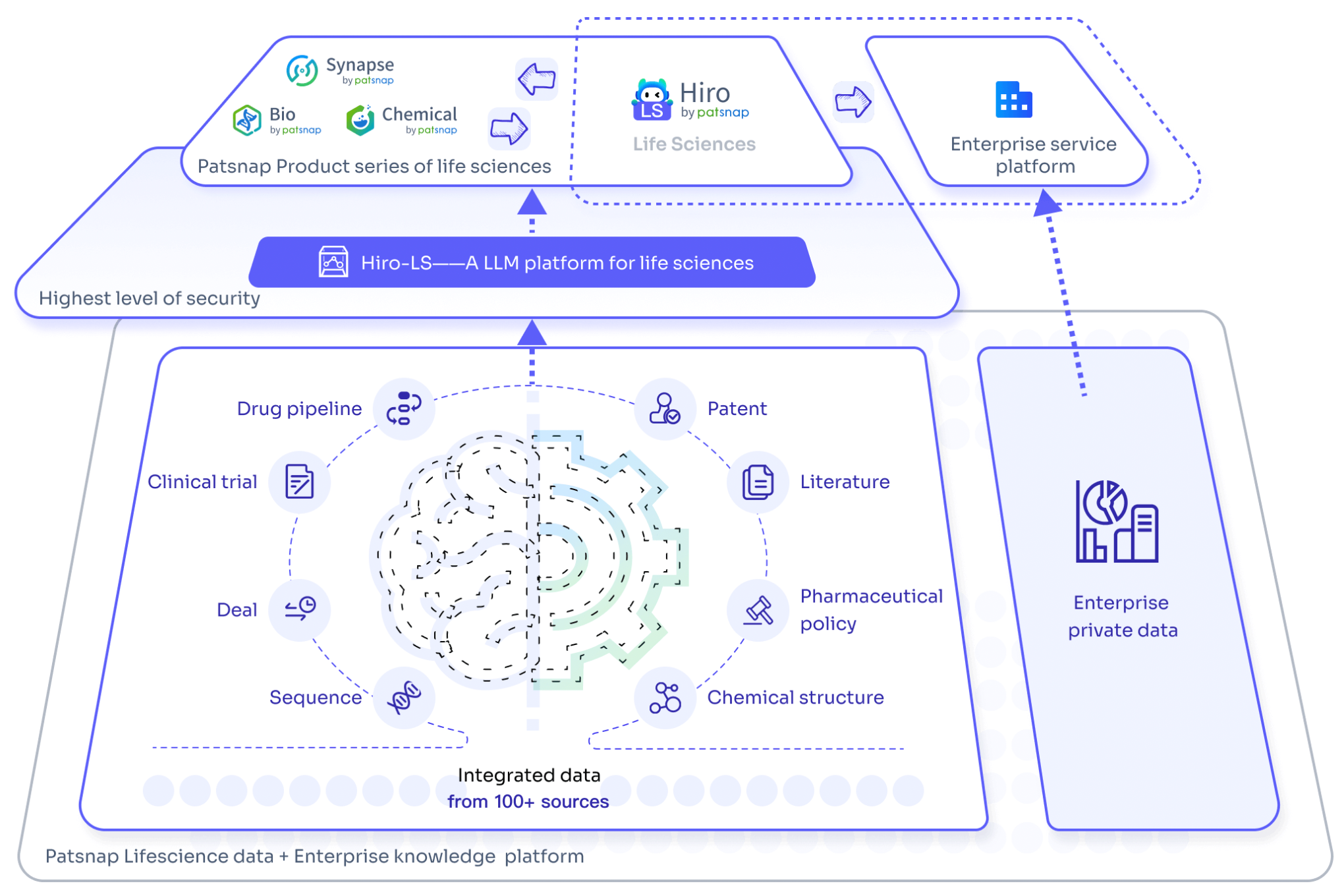