Paclitaxel, a chemotherapeutic agent widely used in the treatment of various
cancers, operates through a unique mechanism that targets the microtubule network within cells. The primary action of Paclitaxel is to stabilize microtubules, which are essential components of the cell's cytoskeleton and play a critical role in cell division, intracellular transport, and maintaining cell shape.
Microtubules are dynamic structures composed of
alpha-tubulin and
beta-tubulin protein subunits. In a normal cell cycle, microtubules undergo continuous phases of polymerization and depolymerization, a process crucial for the proper segregation of chromosomes during mitosis. By interfering with this dynamic equilibrium, Paclitaxel effectively disrupts cell division, which is particularly detrimental to rapidly dividing cancer cells.
Upon administration, Paclitaxel binds to the beta-tubulin subunit of microtubules. This binding promotes the polymerization of
tubulin into stable microtubules and simultaneously inhibits their depolymerization. As a result, microtubules become excessively stabilized and resistant to the normal disassembly processes required for mitosis. Consequently, the formation of stable, non-functional microtubule bundles interferes with the mitotic spindle formation, leading to cell cycle arrest at the G2/M phase. Cells are unable to proceed through mitosis, resulting in apoptosis or programmed cell death.
The ability of Paclitaxel to induce mitotic arrest and apoptosis is particularly effective against cancer cells due to their high rates of division and growth. However, this mechanism also affects normal cells, which can lead to the side effects commonly associated with Paclitaxel, such as
neuropathy,
myelosuppression, and gastrointestinal disturbances.
In addition to its primary mechanism of action, Paclitaxel has been observed to trigger other cellular pathways that contribute to its antitumor effects. For instance, it can activate various signaling pathways involved in apoptosis, such as the
Bcl-2 family proteins, and can also induce oxidative stress within cancer cells, further promoting cell death.
In clinical settings, Paclitaxel is often formulated with agents that improve its solubility and delivery, such as Cremophor EL. It is administered intravenously and is commonly used in combination with other chemotherapeutic drugs to enhance its efficacy. Despite its potent anticancer activity, the development of resistance to Paclitaxel is a significant challenge. Cancer cells can acquire resistance through various mechanisms, including alterations in tubulin isotypes, overexpression of drug efflux pumps, and changes in apoptotic signaling pathways.
Research is ongoing to develop novel formulations and combination therapies to overcome resistance and improve the therapeutic outcomes of Paclitaxel. Additionally, efforts are being made to identify biomarkers that can predict response to Paclitaxel, allowing for more personalized and effective cancer treatment strategies.
In conclusion, Paclitaxel exerts its antitumor effects primarily through the stabilization of microtubules, leading to mitotic arrest and apoptosis in cancer cells. While highly effective, the complexity of its action and the potential for resistance necessitate ongoing research to optimize its use and improve patient outcomes in cancer therapy.
How to obtain the latest development progress of all drugs?
In the Synapse database, you can stay updated on the latest research and development advances of all drugs. This service is accessible anytime and anywhere, with updates available daily or weekly. Use the "Set Alert" function to stay informed. Click on the image below to embark on a brand new journey of drug discovery!
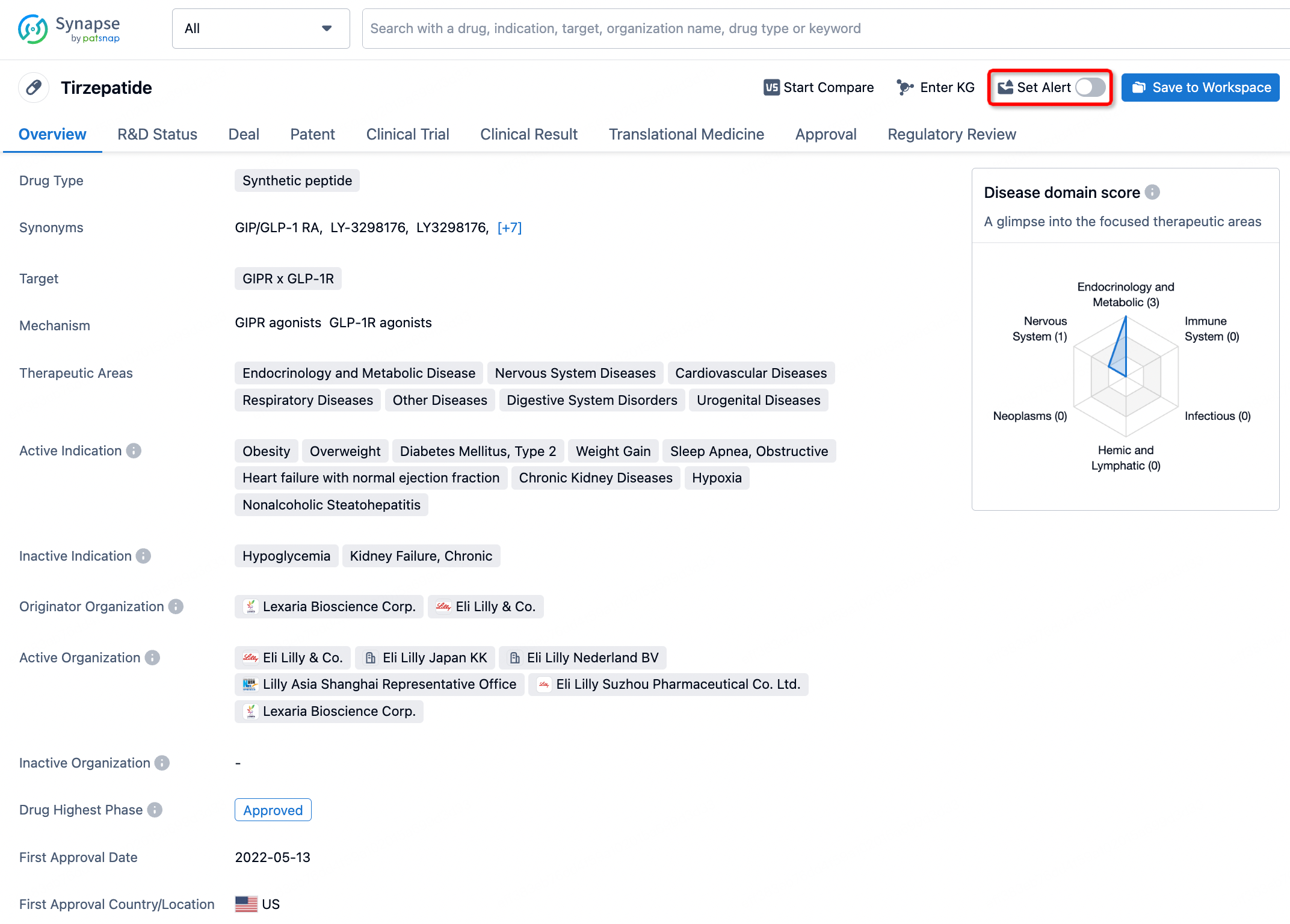
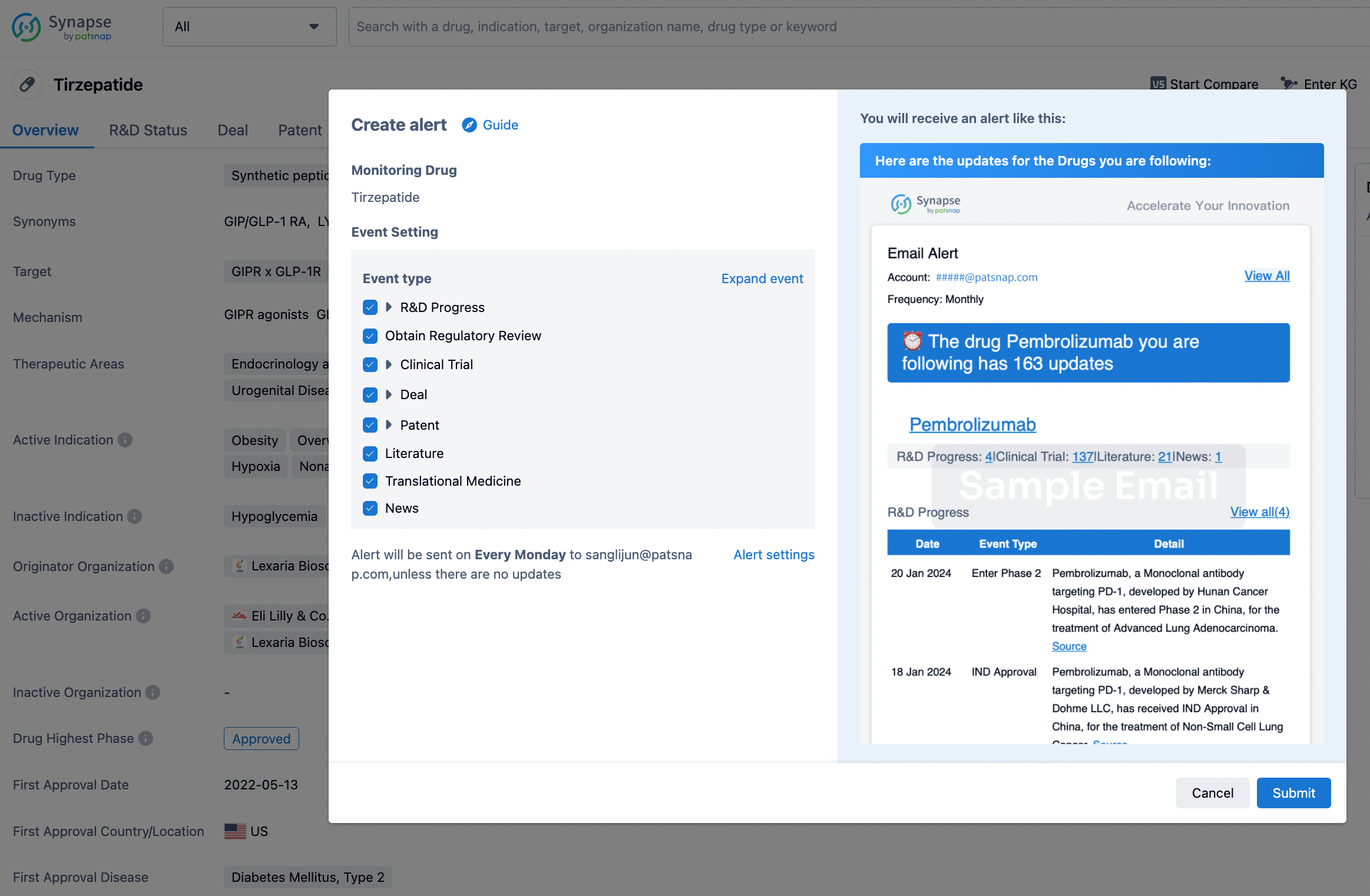
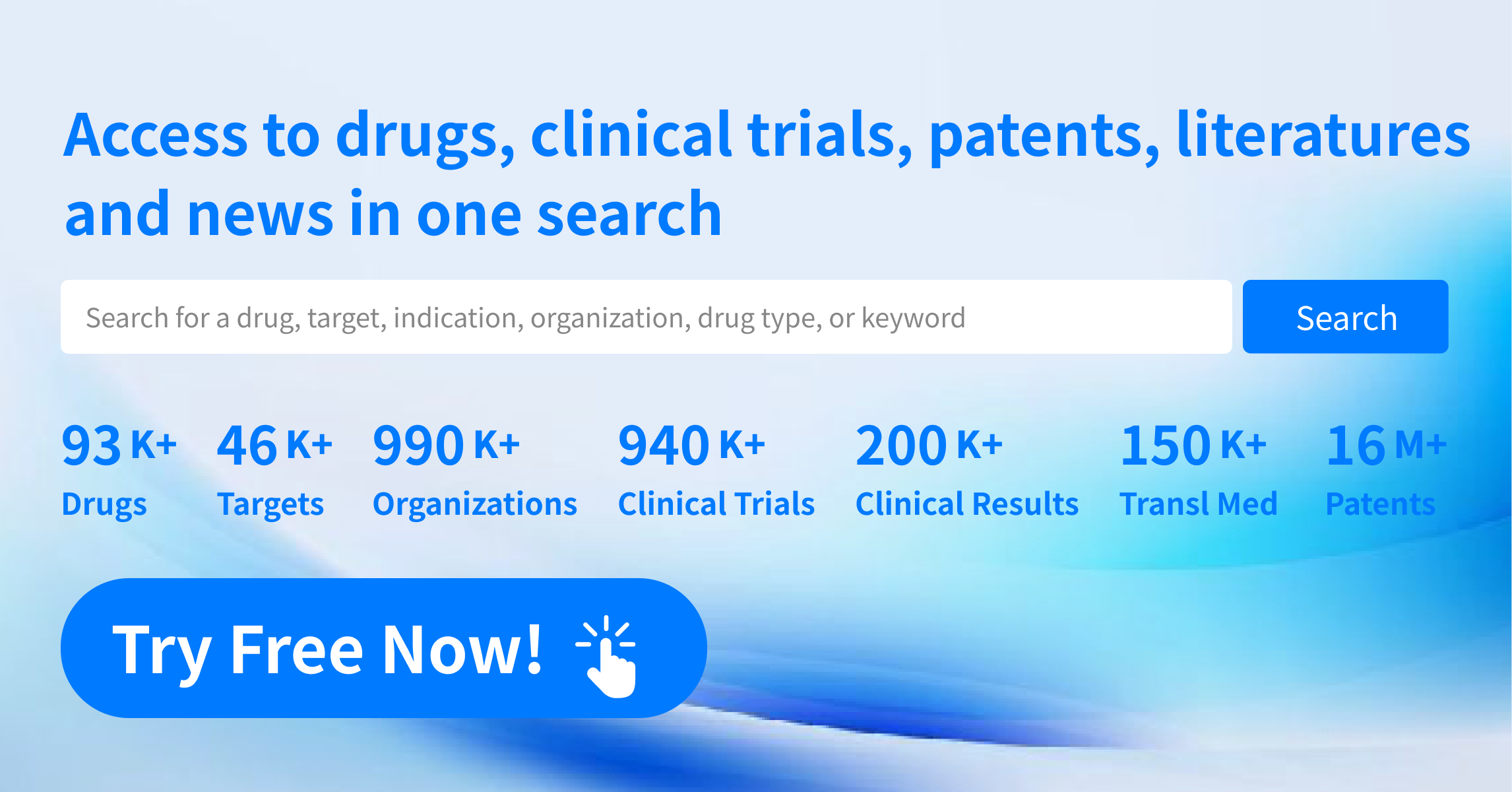